Monocyte differentiation and antigen-presenting functions
Monocytes develop in the bone marrow and represent the primary type of mononuclear phagocyte found in the blood. They were long thought of as a source for tissue macrophages, but recent studies indicate more complex roles for monocytes, both within the circulation and after their migration into tissues and lymphoid organs. In this Review, we discuss the newer concepts underlying the maturation of emigrating monocytes into different classes of tissue macrophages, as well as their potential functions, as monocyte-derived cells, in the tissues. In addition, we consider the emerging roles for monocytes in adaptive immunity as antigen-presenting cells.
This is a preview of subscription content, access via your institution
Access options
Access Nature and 54 other Nature Portfolio journals
Get Nature+, our best-value online-access subscription
cancel any time
Subscribe to this journal
Receive 12 print issues and online access
206,07 € per year
only 17,17 € per issue
Buy this article
- Purchase on SpringerLink
- Instant access to full article PDF
Prices may be subject to local taxes which are calculated during checkout
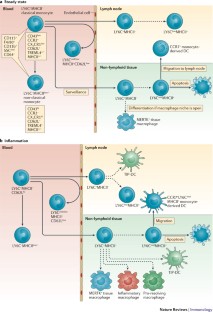
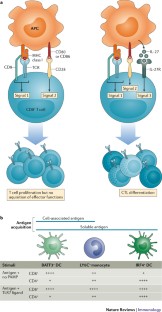
Similar content being viewed by others
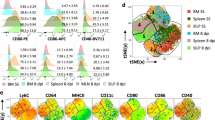
Monocytes undergo multi-step differentiation in mice during oral infection by Toxoplasma gondii
Article Open access 18 December 2019
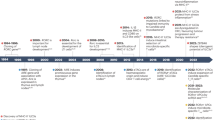
The emerging family of RORγt + antigen-presenting cells
Article 21 July 2023
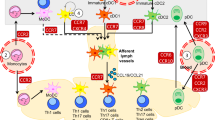
Dendritic cell migration in inflammation and immunity
Article 23 July 2021
References
- Hopkinson-Woolley, J., Hughes, D., Gordon, S. & Martin, P. Macrophage recruitment during limb development and wound healing in the embryonic and foetal mouse. J. Cell Sci.107, 1159–1167 (1994). PubMedGoogle Scholar
- Ginhoux, F. & Jung, S. Monocytes and macrophages: developmental pathways and tissue homeostasis. Nat. Rev. Immunol.14, 392–404 (2014). ArticleCASPubMedGoogle Scholar
- Janssen, W. J. et al. Fas determines differential fates of resident and recruited macrophages during resolution of acute lung injury. Am. J. Respir. Crit. Care Med.184, 547–560 (2011). This manuscript reports that alveolar macrophages are not replenished or replaced by monocytes during inflammation.ArticleCASPubMedPubMed CentralGoogle Scholar
- Yona, S. et al. Fate mapping reveals origins and dynamics of monocytes and tissue macrophages under homeostasis. Immunity38, 79–91 (2013). This study demonstrates that the majority of macrophage populations are established before birth and that short-lived LY6C + monocytes are the obligatory steady-state precursors of blood-resident LY6C−monocytes.ArticleCASPubMedGoogle Scholar
- Sheng, J., Ruedl, C. & Karjalainen, K. Most tissue-resident macrophages except microglia are derived from fetal hematopoietic stem cells. Immunity43, 382–393 (2015). ArticleCASPubMedGoogle Scholar
- Hoeffel, G. et al. c-Myb + erythro-myeloid progenitor-derived fetal monocytes give rise to adult tissue-resident macrophages. Immunity42, 665–678 (2015). ArticleCASPubMedPubMed CentralGoogle Scholar
- Gomez Perdiguero, E. et al. Tissue-resident macrophages originate from yolk-sac-derived erythro-myeloid progenitors. Nature518, 547–551 (2015). ArticlePubMedCASGoogle Scholar
- Haldar, M. & Murphy, K. M. Origin, development, and homeostasis of tissue-resident macrophages. Immunol. Rev.262, 25–35 (2014). ArticleCASPubMedPubMed CentralGoogle Scholar
- Schulz, C. et al. A lineage of myeloid cells independent of Myb and hematopoietic stem cells. Science336, 86–90 (2012). ArticleCASPubMedGoogle Scholar
- Hashimoto, D. et al. Tissue-resident macrophages self-maintain locally throughout adult life with minimal contribution from circulating monocytes. Immunity38, 792–804 (2013). ArticleCASPubMedGoogle Scholar
- Hoeffel, G. et al. Adult Langerhans cells derive predominantly from embryonic fetal liver monocytes with a minor contribution of yolk sac-derived macrophages. J. Exp. Med.209, 1167–1181 (2012). ArticleCASPubMedPubMed CentralGoogle Scholar
- Ginhoux, F. et al. Fate mapping analysis reveals that adult microglia derive from primitive macrophages. Science330, 841–845 (2010). ArticleCASPubMedPubMed CentralGoogle Scholar
- Bain, C. C. et al. Constant replenishment from circulating monocytes maintains the macrophage pool in the intestine of adult mice. Nat. Immunol.15, 929–937 (2014). ArticleCASPubMedPubMed CentralGoogle Scholar
- Ensan, S. et al. Self-renewing resident arterial macrophages arise from embryonic CX3CR1 + precursors and circulating monocytes immediately after birth. Nat. Immunol.17, 159–168 (2016). ArticleCASPubMedGoogle Scholar
- Epelman, S. et al. Embryonic and adult-derived resident cardiac macrophages are maintained through distinct mechanisms at steady state and during inflammation. Immunity40, 91–104 (2014). This manuscript reports the existence of distinct cardiac macrophage populations.ArticleCASPubMedPubMed CentralGoogle Scholar
- Scott, C. L. et al. Bone marrow-derived monocytes give rise to self-renewing and fully differentiated Kupffer cells. Nat. Commun.7, 10321 (2016). ArticleCASPubMedPubMed CentralGoogle Scholar
- Bain, C. C. et al. Long-lived self-renewing bone marrow-derived macrophages displace embryo-derived cells to inhabit adult serous cavities. Nat. Commun.7, ncomms11852 (2016). ArticleCASPubMedPubMed CentralGoogle Scholar
- Jakubzick, C. et al. Minimal differentiation of classical monocytes as they survey steady-state tissues and transport antigen to lymph nodes. Immunity39, 599–610 (2013). This study shows that LY6C + monocytes, and not LY6C−monocytes, constitutively enter tissues and lymph nodes from the blood, and that their interaction with the endothelium promotes their differentiation into MHC class II + monocytes in the steady state. It also shows that LY6C + monocytes survey the tissue environment for antigen acquisition and migrate to the draining lymph nodes.ArticleCASPubMedGoogle Scholar
- Desch, A. N. et al. Flow cytometric analysis of mononuclear phagocytes in non-diseased human lung and lung-draining lymph nodes. Am. J. Respir. Crit. Care Med.193, 614–626 (2016). This study shows that humans have at least as many monocytes as DCs in the lungs and lung-draining lymph nodes.ArticleCASPubMedPubMed CentralGoogle Scholar
- Kim, T. S. & Braciale, T. J. Respiratory dendritic cell subsets differ in their capacity to support the induction of virus-specific cytotoxic CD8 + T cell responses. PLoS ONE4, e4204 (2009). This manuscript reports that monocytes migrate to the draining lymph nodes after infection and present antigen to T cells.ArticlePubMedPubMed CentralCASGoogle Scholar
- Zigmond, E. et al. Ly6C hi monocytes in the inflamed colon give rise to proinflammatory effector cells and migratory antigen-presenting cells. Immunity37, 1076–1090 (2012). ArticleCASPubMedGoogle Scholar
- Cheong, C. et al. Microbial stimulation fully differentiates monocytes to DC-SIGN/CD209 + dendritic cells for immune T cell areas. Cell143, 416–429 (2010). ArticleCASPubMedPubMed CentralGoogle Scholar
- Hohl, T. M. et al. Inflammatory monocytes facilitate adaptive CD4 T cell responses during respiratory fungal infection. Cell Host Microbe6, 470–481 (2009). ArticleCASPubMedPubMed CentralGoogle Scholar
- Sponaas, A. M. et al. Migrating monocytes recruited to the spleen play an important role in control of blood stage malaria. Blood114, 5522–5531 (2009). ArticleCASPubMedGoogle Scholar
- Flores-Langarica, A. et al. T-Zone localized monocyte-derived dendritic cells promote Th1 priming to Salmonella. Eur. J. Immunol.41, 2654–2665 (2011). ArticleCASPubMedGoogle Scholar
- Langlet, C. et al. CD64 expression distinguishes monocyte-derived and conventional dendritic cells and reveals their distinct role during intramuscular immunization. J. Immunol.188, 1751–1760 (2012). ArticleCASPubMedGoogle Scholar
- Nakano, H. et al. Blood-derived inflammatory dendritic cells in lymph nodes stimulate acute T helper type 1 immune responses. Nat. Immunol.10, 394–402 (2009). This manuscript reports that monocytes contribute to the development of TH1 responses during viral infection.ArticleCASPubMedPubMed CentralGoogle Scholar
- Plantinga, M. et al. Conventional and monocyte-derived CD11b + dendritic cells initiate and maintain T helper 2 cell-mediated immunity to house dust mite allergen. Immunity38, 322–335 (2013). ArticleCASPubMedGoogle Scholar
- Shi, C. & Pamer, E. G. Monocyte recruitment during infection and inflammation. Nat. Rev. Immunol.11, 762–774 (2011). ArticleCASPubMedPubMed CentralGoogle Scholar
- Augier, S. et al. Inflammatory blood monocytes contribute to tumor development and represent a privileged target to improve host immunosurveillance. J. Immunol.185, 7165–7173 (2010). ArticleCASPubMedGoogle Scholar
- Epelman, S., Lavine, K. J. & Randolph, G. J. Origin and functions of tissue macrophages. Immunity41, 21–35 (2014). ArticleCASPubMedPubMed CentralGoogle Scholar
- Gautier, E. L., Jakubzick, C. & Randolph, G. J. Regulation of the migration and survival of monocyte subsets by chemokine receptors and its relevance to atherosclerosis. Arterioscler. Thromb. Vasc. Biol.29, 1412–1418 (2009). ArticleCASPubMedPubMed CentralGoogle Scholar
- Ingersoll, M. A., Platt, A. M., Potteaux, S. & Randolph, G. J. Monocyte trafficking in acute and chronic inflammation. Trends Immunol.32, 470–477 (2011). ArticleCASPubMedPubMed CentralGoogle Scholar
- Randolph, G. J., Jakubzick, C. & Qu, C. Antigen presentation by monocytes and monocyte-derived cells. Curr. Opin. Immunol.20, 52–60 (2008). ArticleCASPubMedGoogle Scholar
- Hettinger, J. et al. Origin of monocytes and macrophages in a committed progenitor. Nat. Immunol.14, 821–830 (2013). ArticleCASPubMedGoogle Scholar
- Auffray, C. et al. Monitoring of blood vessels and tissues by a population of monocytes with patrolling behavior. Science317, 666–670 (2007). ArticleCASPubMedGoogle Scholar
- Ingersoll, M. A. et al. Comparison of gene expression profiles between human and mouse monocyte subsets. Blood115, e10–e19 (2010). ArticleCASPubMedPubMed CentralGoogle Scholar
- Randolph, G. J., Beaulieu, S., Lebecque, S., Steinman, R. M. & Muller, W. A. Differentiation of monocytes into dendritic cells in a model of transendothelial trafficking. Science282, 480–483 (1998). ArticleCASPubMedGoogle Scholar
- Thomas, G. D. et al. Deleting an Nr4a1 super-enhancer subdomain ablates Ly6C low monocytes while preserving macrophage gene function. Immunity45, 975–987 (2016). ArticleCASPubMedPubMed CentralGoogle Scholar
- Hanna, R. N. et al. The transcription factor NR4A1 (Nur77) controls bone marrow differentiation and the survival of Ly6C − monocytes. Nat. Immunol.12, 778–785 (2011). ArticleCASPubMedPubMed CentralGoogle Scholar
- Gamrekelashvili, J. et al. Regulation of monocyte cell fate by blood vessels mediated by Notch signalling. Nat. Commun.7, 12597 (2016). ArticleCASPubMedPubMed CentralGoogle Scholar
- Landsman, L. et al. CX3CR1 is required for monocyte homeostasis and atherogenesis by promoting cell survival. Blood113, 963–972 (2009). ArticleCASPubMedGoogle Scholar
- Jakubzick, C. et al. Blood monocyte subsets differentially give rise to CD103 + and CD103 − pulmonary dendritic cell populations. J. Immunol.180, 3019–3027 (2008). ArticleCASPubMedGoogle Scholar
- Tamoutounour, S. et al. Origins and functional specialization of macrophages and of conventional and monocyte-derived dendritic cells in mouse skin. Immunity39, 925–938 (2013). This study demonstrates that LY6C + monocytes give rise to MHC class II + tissue monocytes that either remain in tissue or traffic to the draining lymph nodes. It also identifies dermal interstitial macrophages.ArticleCASPubMedGoogle Scholar
- Serbina, N. V., Salazar-Mather, T. P., Biron, C. A., Kuziel, W. A. & Pamer, E. G. TNF/iNOS-producing dendritic cells mediate innate immune defense against bacterial infection. Immunity19, 59–70 (2003). This manuscript reports that LY6C + monocytes express high levels of TNF and iNOS during a bacterial infection.ArticleCASPubMedGoogle Scholar
- Samstein, M. et al. Essential yet limited role for CCR2 + inflammatory monocytes during Mycobacterium tuberculosis-specific T cell priming. eLife2, e01086 (2013). ArticlePubMedPubMed CentralCASGoogle Scholar
- Rivollier, A., He, J., Kole, A., Valatas, V. & Kelsall, B. L. Inflammation switches the differentiation program of Ly6C hi monocytes from antiinflammatory macrophages to inflammatory dendritic cells in the colon. J. Exp. Med.209, 139–155 (2012). ArticleCASPubMedPubMed CentralGoogle Scholar
- Bain, C. C. et al. Resident and pro-inflammatory macrophages in the colon represent alternative context-dependent fates of the same Ly6C hi monocyte precursors. Mucosal Immunol.6, 498–510 (2013). ArticleCASPubMedGoogle Scholar
- Liu, K. et al. Origin of dendritic cells in peripheral lymphoid organs of mice. Nat. Immunol.8, 578–583 (2007). This manuscript reports that circulating LY6C + monocytes do not reach equilibrium in parabiotic mice.ArticleCASPubMedGoogle Scholar
- Tsou, C. L. et al. Critical roles for CCR2 and MCP-3 in monocyte mobilization from bone marrow and recruitment to inflammatory sites. J. Clin. Invest.117, 902–909 (2007). ArticleCASPubMedPubMed CentralGoogle Scholar
- Serbina, N. V. & Pamer, E. G. Monocyte emigration from bone marrow during bacterial infection requires signals mediated by chemokine receptor CCR2. Nat. Immunol.7, 311–317 (2006). This study shows that LY6C + monocytes need CCR2 to exit the bone marrow.ArticleCASPubMedGoogle Scholar
- Palframan, R. T. et al. Inflammatory chemokine transport and presentation in HEV: a remote control mechanism for monocyte recruitment to lymph nodes in inflamed tissues. J. Exp. Med.194, 1361–1373 (2001). ArticleCASPubMedPubMed CentralGoogle Scholar
- Qu, C. et al. Role of CCR8 and other chemokine pathways in the migration of monocyte-derived dendritic cells to lymph nodes. J. Exp. Med.200, 1231–1241 (2004). ArticleCASPubMedPubMed CentralGoogle Scholar
- Gautier, E. L., Ivanov, S., Lesnik, P. & Randolph, G. J. Local apoptosis mediates clearance of macrophages from resolving inflammation in mice. Blood122, 2714–2722 (2013). ArticleCASPubMedPubMed CentralGoogle Scholar
- Doerschuk, C. M. Neutrophil rheology and transit through capillaries and sinusoids. Am. J. Respir. Crit. Care Med.159, 1693–1695 (1999). ArticleCASPubMedGoogle Scholar
- Doerschuk, C. M. et al. Leukocyte and platelet margination within microvasculature of rabbit lungs. J. Appl. Physiol.68, 1956–1961 (1990). ArticleCASPubMedGoogle Scholar
- Vandivier, R. W., Henson, P. M. & Douglas, I. S. Burying the dead: the impact of failed apoptotic cell removal (efferocytosis) on chronic inflammatory lung disease. Chest129, 1673–1682 (2006). ArticlePubMedGoogle Scholar
- Kratofil, R. M., Kubes, P. & Deniset, J. F. Monocyte conversion during inflammation and injury. Arterioscler. Thromb. Vasc. Biol.37, 35–42 (2017). ArticleCASPubMedGoogle Scholar
- Dal-Secco, D. et al. A dynamic spectrum of monocytes arising from the in situ reprogramming of CCR2 + monocytes at a site of sterile injury. J. Exp. Med.212, 447–456 (2015). ArticleCASPubMedPubMed CentralGoogle Scholar
- Gautier, E. L. et al. Gene-expression profiles and transcriptional regulatory pathways that underlie the identity and diversity of mouse tissue macrophages. Nat. Immunol.13, 1118–1128 (2012). This study highlights the gene transcripts that are distinctly and universally expressed among tissue-specific macrophages. It also identifies cell-surface proteins that help to distinguish macrophages from DCs and monocytes.ArticleCASPubMedPubMed CentralGoogle Scholar
- Rantakari, P. et al. Fetal liver endothelium regulates the seeding of tissue-resident macrophages. Nature538, 392–396 (2016). ArticleCASPubMedGoogle Scholar
- Tamoutounour, S. et al. CD64 distinguishes macrophages from dendritic cells in the gut and reveals the Th1-inducing role of mesenteric lymph node macrophages during colitis. Eur. J. Immunol.42, 3150–3166 (2012). This study identifies distinct macrophage populations in the gut.ArticleCASPubMedGoogle Scholar
- Gibbings, S. L. et al. Three unique interstitial macrophages in the murine lung at steady state. Am. J. Respir. Cell Mol. Biol.http://dx.doi.org/10.1165/rcmb.2016-0361OC (2017). This study identifies three unique interstitial macrophage populations in the lung that are also present in other organs such as the heart, gut and skin.
- Gibbings, S. L. et al. Transcriptome analysis highlights the conserved difference between embryonic and postnatal-derived alveolar macrophages. Blood126, 1357–1366 (2015). This study shows that the development of alveolar macrophages is largely dictated by the environment rather than by the origin of the cell; however, some genes linked to cell origin are not altered by the environment, such asMarcofor embryonically derived alveolar macrophages, andC1qandPlbd1(which encodes phospholipase B domain-containing protein 1) for postnatally derived alveolar macrophages.ArticleCASPubMedPubMed CentralGoogle Scholar
- Scott, C. L., Henri, S. & Guilliams, M. Mononuclear phagocytes of the intestine, the skin, and the lung. Immunol. Rev.262, 9–24 (2014). ArticleCASPubMedGoogle Scholar
- Okabe, Y. & Medzhitov, R. Tissue biology perspective on macrophages. Nat. Immunol.17, 9–17 (2016). ArticleCASPubMedGoogle Scholar
- van de Laar, L. et al. Yolk sac macrophages, fetal liver, and adult monocytes can colonize an empty niche and develop into functional tissue-resident macrophages. Immunity44, 755–768 (2016). ArticleCASPubMedGoogle Scholar
- Lavin, Y. et al. Tissue-resident macrophage enhancer landscapes are shaped by the local microenvironment. Cell159, 1312–1326 (2014). ArticleCASPubMedPubMed CentralGoogle Scholar
- Jantsch, J. et al. Cutaneous Na + storage strengthens the antimicrobial barrier function of the skin and boosts macrophage-driven host defense. Cell Metab.21, 493–501 (2015). ArticleCASPubMedPubMed CentralGoogle Scholar
- Stamatiades, E. G. et al. Immune monitoring of trans-endothelial transport by kidney-resident macrophages. Cell166, 991–1003 (2016). ArticleCASPubMedPubMed CentralGoogle Scholar
- Theurl, I. et al. On-demand erythrocyte disposal and iron recycling requires transient macrophages in the liver. Nat. Med.22, 945–951 (2016). ArticleCASPubMedPubMed CentralGoogle Scholar
- Menezes, S. et al. The heterogeneity of Ly6C hi monocytes controls their differentiation into iNOS + macrophages or monocyte-derived dendritic cells. Immunity45, 1205–1218 (2016). ArticleCASPubMedPubMed CentralGoogle Scholar
- Ginhoux, F., Schultze, J. L., Murray, P. J., Ochando, J. & Biswas, S. K. New insights into the multidimensional concept of macrophage ontogeny, activation and function. Nat. Immunol.17, 34–40 (2016). ArticleCASPubMedGoogle Scholar
- Hoeffel, G. & Ginhoux, F. Ontogeny of tissue-resident macrophages. Front. Immunol.6, 486 (2015). ArticlePubMedPubMed CentralCASGoogle Scholar
- Perdiguero, E. G. & Geissmann, F. The development and maintenance of resident macrophages. Nat. Immunol.17, 2–8 (2016). ArticleCASPubMedPubMed CentralGoogle Scholar
- Ginhoux, F. & Guilliams, M. Tissue-resident macrophage ontogeny and homeostasis. Immunity44, 439–449 (2016). ArticleCASPubMedGoogle Scholar
- Goldszmid, R. S. et al. NK cell-derived interferon-γ orchestrates cellular dynamics and the differentiation of monocytes into dendritic cells at the site of infection. Immunity36, 1047–1059 (2012). ArticleCASPubMedPubMed CentralGoogle Scholar
- Robben, P. M., LaRegina, M., Kuziel, W. A. & Sibley, L. D. Recruitment of Gr-1 + monocytes is essential for control of acute toxoplasmosis. J. Exp. Med.201, 1761–1769 (2005). ArticleCASPubMedPubMed CentralGoogle Scholar
- Zhu, B. et al. Plasticity of Ly-6C hi myeloid cells in T cell regulation. J. Immunol.187, 2418–2432 (2011). ArticleCASPubMedGoogle Scholar
- Leon, B., Lopez-Bravo, M. & Ardavin, C. Monocyte-derived dendritic cells formed at the infection site control the induction of protective T helper 1 responses against Leishmania. Immunity26, 519–531 (2007). ArticleCASPubMedGoogle Scholar
- Steinman, R. M. & Witmer, M. D. Lymphoid dendritic cells are potent stimulators of the primary mixed leukocyte reaction in mice. Proc. Natl Acad. Sci. USA75, 5132–5136 (1978). ArticleCASPubMedPubMed CentralGoogle Scholar
- Jung, S. et al. In vivo depletion of CD11c + dendritic cells abrogates priming of CD8 + T cells by exogenous cell-associated antigens. Immunity17, 211–220 (2002). ArticleCASPubMedPubMed CentralGoogle Scholar
- van Rijt, L. S. et al. In vivo depletion of lung CD11c + dendritic cells during allergen challenge abrogates the characteristic features of asthma. J. Exp. Med.201, 981–991 (2005). ArticleCASPubMedPubMed CentralGoogle Scholar
- Turley, S. J. et al. Transport of peptide–MHC class II complexes in developing dendritic cells. Science288, 522–527 (2000). ArticleCASPubMedGoogle Scholar
- Delamarre, L., Pack, M., Chang, H., Mellman, I. & Trombetta, E. S. Differential lysosomal proteolysis in antigen-presenting cells determines antigen fate. Science307, 1630–1634 (2005). ArticleCASPubMedGoogle Scholar
- Desch, A. N. et al. Dendritic cell subsets require cis-activation for cytotoxic CD8 T-cell induction. Nat. Commun.5, 4674 (2014). This manuscript reports that thein vivoinduction of cytotoxic T cells requirescis-activation by endogenous DCs (that is, the DC subset that is presenting antigen must be simultaneously stimulated through its pattern recognition receptors).ArticleCASPubMedGoogle Scholar
- Maldonado-Lopez, R. et al. CD8α + and CD8α − subclasses of dendritic cells direct the development of distinct T helper cells in vivo. J. Exp. Med.189, 587–592 (1999). ArticleCASPubMedPubMed CentralGoogle Scholar
- Le Bon, A. et al. Cross-priming of CD8 + T cells stimulated by virus-induced type I interferon. Nat. Immunol.4, 1009–1015 (2003). ArticleCASPubMedGoogle Scholar
- Williams, J. W. et al. Transcription factor IRF4 drives dendritic cells to promote Th2 differentiation. Nat. Commun.4, 2990 (2013). ArticlePubMedCASGoogle Scholar
- Schlitzer, A. et al. IRF4 transcription factor-dependent CD11b + dendritic cells in human and mouse control mucosal IL-17 cytokine responses. Immunity38, 970–983 (2013). ArticleCASPubMedPubMed CentralGoogle Scholar
- GeurtsvanKessel, C. H. et al. Clearance of influenza virus from the lung depends on migratory langerin + CD11b − but not plasmacytoid dendritic cells. J. Exp. Med.205, 1621–1634 (2008). ArticleCASPubMedPubMed CentralGoogle Scholar
- Belz, G. T. et al. CD36 is differentially expressed by CD8 + splenic dendritic cells but is not required for cross-presentation in vivo. J. Immunol.168, 6066–6070 (2002). ArticleCASPubMedGoogle Scholar
- Idoyaga, J. et al. Comparable T helper 1 (Th1) and CD8 T-cell immunity by targeting HIV gag p24 to CD8 dendritic cells within antibodies to langerin, DEC205, and Clec9A. Proc. Natl Acad. Sci. USA108, 2384–2389 (2011). ArticleCASPubMedPubMed CentralGoogle Scholar
- Edelson, B. T. et al. Peripheral CD103 + dendritic cells form a unified subset developmentally related to CD8α + conventional dendritic cells. J. Exp. Med.207, 823–836 (2010). ArticleCASPubMedPubMed CentralGoogle Scholar
- Pulko, V. et al. TLR3-stimulated dendritic cells up-regulate B7-H1 expression and influence the magnitude of CD8 T cell responses to tumor vaccination. J. Immunol.183, 3634–3641 (2009). ArticleCASPubMedGoogle Scholar
- Belz, G. T. et al. Cutting edge: conventional CD8α + dendritic cells are generally involved in priming CTL immunity to viruses. J. Immunol.172, 1996–2000 (2004). ArticleCASPubMedGoogle Scholar
- Allan, R. S. et al. Epidermal viral immunity induced by CD8α + dendritic cells but not by Langerhans cells. Science301, 1925–1928 (2003). ArticleCASPubMedGoogle Scholar
- Iyoda, T. et al. The CD8 + dendritic cell subset selectively endocytoses dying cells in culture and in vivo. J. Exp. Med.195, 1289–1302 (2002). ArticleCASPubMedPubMed CentralGoogle Scholar
- Pooley, J. L., Heath, W. R. & Shortman, K. Cutting edge: intravenous soluble antigen is presented to CD4 T cells by CD8 − dendritic cells, but cross-presented to CD8 T cells by CD8 + dendritic cells. J. Immunol.166, 5327–5330 (2001). ArticleCASPubMedGoogle Scholar
- del Rio, M. L., Rodriguez-Barbosa, J. I., Kremmer, E. & Forster, R. CD103 − and CD103 + bronchial lymph node dendritic cells are specialized in presenting and cross-presenting innocuous antigen to CD4 + and CD8 + T cells. J. Immunol.178, 6861–6866 (2007). ArticleCASPubMedGoogle Scholar
- den Haan, J. M., Lehar, S. M. & Bevan, M. J. CD8 + but not CD8 − dendritic cells cross-prime cytotoxic T cells in vivo. J. Exp. Med.192, 1685–1696 (2000). ArticleCASPubMedPubMed CentralGoogle Scholar
- Dudziak, D. et al. Differential antigen processing by dendritic cell subsets in vivo. Science315, 107–111 (2007). ArticleCASPubMedGoogle Scholar
- Kim, T. S., Gorski, S. A., Hahn, S., Murphy, K. M. & Braciale, T. J. Distinct dendritic cell subsets dictate the fate decision between effector and memory CD8 + T cell differentiation by a CD24-dependent mechanism. Immunity40, 400–413 (2014). ArticleCASPubMedPubMed CentralGoogle Scholar
- Desch, A. N., Henson, P. M. & Jakubzick, C. V. Pulmonary dendritic cell development and antigen acquisition. Immunol. Res.55, 178–186 (2013). ArticleCASPubMedPubMed CentralGoogle Scholar
- Desch, A. N. et al. CD103 + pulmonary dendritic cells preferentially acquire and present apoptotic cell-associated antigen. J. Exp. Med.208, 1789–1797 (2011). ArticleCASPubMedPubMed CentralGoogle Scholar
- Jakubzick, C., Helft, J., Kaplan, T. J. & Randolph, G. J. Optimization of methods to study pulmonary dendritic cell migration reveals distinct capacities of DC subsets to acquire soluble versus particulate antigen. J. Immunol. Methods337, 121–131 (2008). ArticleCASPubMedPubMed CentralGoogle Scholar
- Atif, S. M. et al. Cutting edge: roles for Batf3-dependent APCs in the rejection of minor histocompatibility antigen-mismatched grafts. J. Immunol.195, 46–50 (2015). ArticleCASPubMedGoogle Scholar
- Ferris, S. T. et al. A minor subset of Batf3-dependent antigen-presenting cells in islets of Langerhans is essential for the development of autoimmune diabetes. Immunity41, 657–669 (2014). ArticleCASPubMedPubMed CentralGoogle Scholar
- Iborra, S. et al. Optimal generation of tissue-resident but not circulating memory T cells during viral infection requires crosspriming by DNGR-1 + dendritic cells. Immunity45, 847–860 (2016). ArticleCASPubMedPubMed CentralGoogle Scholar
- Kastenmuller, K. et al. Protective T cell immunity in mice following protein-TLR7/8 agonist-conjugate immunization requires aggregation, type I IFN, and multiple DC subsets. J. Clin. Invest.121, 1782–1796 (2011). ArticleCASPubMedPubMed CentralGoogle Scholar
- Oh, J. Z., Kurche, J. S., Burchill, M. A. & Kedl, R. M. TLR7 enables cross-presentation by multiple dendritic cell subsets through a type I IFN-dependent pathway. Blood118, 3028–3038 (2011). References 110 and 111 show that a TLR7-based adjuvant can stimulate multiple DC subsets, including Langerhans cells, to cross-present antigens to CD8 + T cells.ArticleCASPubMedPubMed CentralGoogle Scholar
- Faure-Andre, G. et al. Regulation of dendritic cell migration by CD74, the MHC class II-associated invariant chain. Science322, 1705–1710 (2008). ArticleCASPubMedGoogle Scholar
- Leon, B. & Ardavin, C. Monocyte migration to inflamed skin and lymph nodes is differentially controlled by L-selectin and PSGL-1. Blood111, 3126–3130 (2008). ArticleCASPubMedGoogle Scholar
- Kamphorst, A. O., Guermonprez, P., Dudziak, D. & Nussenzweig, M. C. Route of antigen uptake differentially impacts presentation by dendritic cells and activated monocytes. J. Immunol.185, 3426–3435 (2010). ArticleCASPubMedGoogle Scholar
- Schreiber, H. A. et al. Intestinal monocytes and macrophages are required for T cell polarization in response to Citrobacter rodentium. J. Exp. Med.210, 2025–2039 (2013). ArticleCASPubMedPubMed CentralGoogle Scholar
- Ersland, K., Wuthrich, M. & Klein, B. S. Dynamic interplay among monocyte-derived, dermal, and resident lymph node dendritic cells during the generation of vaccine immunity to fungi. Cell Host Microbe7, 474–487 (2010). ArticleCASPubMedPubMed CentralGoogle Scholar
- Larson, S. R. et al. Ly6C + monocyte efferocytosis and cross-presentation of cell-associated antigens. Cell Death Differ.23, 997–1003 (2016). This study demonstrates that monocytes are efferocytic and cross-present antigen, features that are similar to those of BATF3 + DCs. It also shows that cross-presentation is enhanced in the presence of a TLR7 ligand, but not a TLR3 or TLR4 ligand.ArticleCASPubMedPubMed CentralGoogle Scholar
- Ko, H. J. et al. GM-CSF-responsive monocyte-derived dendritic cells are pivotal in Th17 pathogenesis. J. Immunol.192, 2202–2209 (2014). ArticleCASPubMedGoogle Scholar
- Yi, H., Guo, C., Yu, X., Zuo, D. & Wang, X. Y. Mouse CD11b + Gr-1 + myeloid cells can promote Th17 cell differentiation and experimental autoimmune encephalomyelitis. J. Immunol.189, 4295–4304 (2012). ArticleCASPubMedGoogle Scholar
- Croxford, A. L. et al. The cytokine GM-CSF drives the inflammatory signature of CCR2 + monocytes and licenses autoimmunity. Immunity43, 502–514 (2015). ArticleCASPubMedGoogle Scholar
- Chow, K. V., Lew, A. M., Sutherland, R. M. & Zhan, Y. Monocyte-derived dendritic cells promote Th polarization, whereas conventional dendritic cells promote Th proliferation. J. Immunol.196, 624–636 (2016). ArticleCASPubMedGoogle Scholar
- Slaney, C. Y., Toker, A., La Flamme, A., Backstrom, B. T. & Harper, J. L. Naive blood monocytes suppress T-cell function. A possible mechanism for protection from autoimmunity. Immunol. Cell Biol.89, 7–13 (2011). ArticleCASPubMedGoogle Scholar
- Mitchell, L. A., Henderson, A. J. & Dow, S. W. Suppression of vaccine immunity by inflammatory monocytes. J. Immunol.189, 5612–5621 (2012). ArticleCASPubMedGoogle Scholar
- Lopez-Bravo, M. et al. IL-4 blocks TH1-polarizing/inflammatory cytokine gene expression during monocyte-derived dendritic cell differentiation through histone hypoacetylation. J. Allergy Clin. Immunol.132, 1409–1419 (2013). ArticleCASPubMedGoogle Scholar
- Iwata, A. et al. Th2-type inflammation instructs inflammatory dendritic cells to induce airway hyperreactivity. Int. Immunol.26, 103–114 (2014). ArticleCASPubMedGoogle Scholar
- Norimoto, A. et al. Dectin-2 promotes house dust mite-induced T helper type 2 and type 17 cell differentiation and allergic airway inflammation in mice. Am. J. Respir. Cell Mol. Biol.51, 201–209 (2014). PubMedGoogle Scholar
- Randolph, G. J., Inaba, K., Robbiani, D. F., Steinman, R. M. & Muller, W. A. Differentiation of phagocytic monocytes into lymph node dendritic cells in vivo. Immunity11, 753–761 (1999). ArticleCASPubMedGoogle Scholar
- Chakarov, S. & Fazilleau, N. Monocyte-derived dendritic cells promote T follicular helper cell differentiation. EMBO Mol. Med.6, 590–603 (2014). ArticleCASPubMedPubMed CentralGoogle Scholar
- Fillatreau, S. Monocyte-derived dendritic cells identified as booster of T follicular helper cell differentiation. EMBO Mol. Med.6, 574–576 (2014). ArticleCASPubMedPubMed CentralGoogle Scholar
- Choi, Y. S. et al. ICOS receptor instructs T follicular helper cell versus effector cell differentiation via induction of the transcriptional repressor Bcl6. Immunity34, 932–946 (2011). ArticleCASPubMedPubMed CentralGoogle Scholar
- Deenick, E. K. et al. Follicular helper T cell differentiation requires continuous antigen presentation that is independent of unique B cell signaling. Immunity33, 241–253 (2010). ArticleCASPubMedPubMed CentralGoogle Scholar
- Goenka, R. et al. Cutting edge: dendritic cell-restricted antigen presentation initiates the follicular helper T cell program but cannot complete ultimate effector differentiation. J. Immunol.187, 1091–1095 (2011). ArticleCASPubMedGoogle Scholar
- Lahoud, M. H. et al. Targeting antigen to mouse dendritic cells via Clec9A induces potent CD4 T cell responses biased toward a follicular helper phenotype. J. Immunol.187, 842–850 (2011). ArticleCASPubMedGoogle Scholar
- Poholek, A. C. et al. In vivo regulation of Bcl6 and T follicular helper cell development. J. Immunol.185, 313–326 (2010). ArticleCASPubMedGoogle Scholar
- Shin, C. et al. CD8α − dendritic cells induce antigen-specific T follicular helper cells generating efficient humoral immune responses. Cell Rep.11, 1929–1940 (2015). ArticleCASPubMedGoogle Scholar
- Kato, Y. et al. Targeting antigen to Clec9A primes follicular Th cell memory responses capable of robust recall. J. Immunol.195, 1006–1014 (2015). ArticleCASPubMedGoogle Scholar
- Dahlgren, M. W. et al. T follicular helper, but not Th1, cell differentiation in the absence of conventional dendritic cells. J. Immunol.194, 5187–5199 (2015). ArticleCASPubMedGoogle Scholar
- Ballesteros-Tato, A. et al. T follicular helper cell plasticity shapes pathogenic T helper 2 cell-mediated immunity to inhaled house dust mite. Immunity44, 259–273 (2016). ArticleCASPubMedPubMed CentralGoogle Scholar
- Guilliams, M., Bruhns, P., Saeys, Y., Hammad, H. & Lambrecht, B. N. The function of Fcγ receptors in dendritic cells and macrophages. Nat. Rev. Immunol.14, 94–108 (2014). ArticleCASPubMedGoogle Scholar
- Segura, E. & Amigorena, S. Cross-presentation by human dendritic cell subsets. Immunol. Lett.158, 73–78 (2014). ArticleCASPubMedGoogle Scholar
- Segura, E. & Amigorena, S. Cross-presentation in mouse and human dendritic cells. Adv. Immunol.127, 1–31 (2015). ArticleCASPubMedGoogle Scholar
- Bedoui, S. et al. Cross-presentation of viral and self antigens by skin-derived CD103 + dendritic cells. Nat. Immunol.10, 488–495 (2009). This study demonstrates the acquisition of virus-infected dying cells and cross-presentation by BATF3 + DCsin vivo. ArticleCASPubMedGoogle Scholar
- Joffre, O. P., Segura, E., Savina, A. & Amigorena, S. Cross-presentation by dendritic cells. Nat. Rev. Immunol.12, 557–569 (2012). ArticleCASPubMedGoogle Scholar
- Lin, M. L. et al. Selective suicide of cross-presenting CD8 + dendritic cells by cytochrome c injection shows functional heterogeneity within this subset. Proc. Natl Acad. Sci. USA105, 3029–3034 (2008). ArticleCASPubMedPubMed CentralGoogle Scholar
- Segura, E. et al. Characterization of resident and migratory dendritic cells in human lymph nodes. J. Exp. Med.209, 653–660 (2012). ArticleCASPubMedPubMed CentralGoogle Scholar
- Qu, C., Nguyen, V. A., Merad, M. & Randolph, G. J. MHC class I/peptide transfer between dendritic cells overcomes poor cross-presentation by monocyte-derived APCs that engulf dying cells. J. Immunol.182, 3650–3659 (2009). ArticleCASPubMedGoogle Scholar
- Kuhn, S., Yang, J. & Ronchese, F. Monocyte-derived dendritic cells are essential for CD8 + T cell activation and antitumor responses after local immunotherapy. Front. Immunol.6, 584 (2015). ArticlePubMedPubMed CentralCASGoogle Scholar
- Carbone, F. R. Tissue-resident memory T cells and fixed immune surveillance in nonlymphoid organs. J. Immunol.195, 17–22 (2015). ArticleCASPubMedGoogle Scholar
- Fogg, D. K. et al. A clonogenic bone marrow progenitor specific for macrophages and dendritic cells. Science311, 83–87 (2006). ArticleCASPubMedGoogle Scholar
- Liu, K. et al. In vivo analysis of dendritic cell development and homeostasis. Science324, 392–397 (2009). ArticleCASPubMedPubMed CentralGoogle Scholar
- Naik, S. H. et al. Development of plasmacytoid and conventional dendritic cell subtypes from single precursor cells derived in vitro and in vivo. Nat. Immunol.8, 1217–1226 (2007). ArticleCASPubMedGoogle Scholar
- Onai, N. et al. Identification of clonogenic common Flt3 + M-CSFR + plasmacytoid and conventional dendritic cell progenitors in mouse bone marrow. Nat. Immunol.8, 1207–1216 (2007). ArticleCASPubMedGoogle Scholar
- Chong, S. Z. et al. CXCR4 identifies transitional bone marrow premonocytes that replenish the mature monocyte pool for peripheral responses. J. Exp. Med.213, 2293–2314 (2016). ArticlePubMedPubMed CentralCASGoogle Scholar
- Swirski, F. K. et al. Identification of splenic reservoir monocytes and their deployment to inflammatory sites. Science325, 612–616 (2009). ArticleCASPubMedPubMed CentralGoogle Scholar
- Ziegler-Heitbrock, H. W. Heterogeneity of human blood monocytes: the CD14 + CD16 + subpopulation. Immunol. Today17, 424–428 (1996). ArticleCASPubMedGoogle Scholar
- Geissmann, F., Jung, S. & Littman, D. R. Blood monocytes consist of two principal subsets with distinct migratory properties. Immunity19, 71–82 (2003). ArticleCASPubMedGoogle Scholar
- Ziegler-Heitbrock, L. et al. Nomenclature of monocytes and dendritic cells in blood. Blood116, e74–e80 (2010). ArticleCASPubMedGoogle Scholar
- Rosenstein, Y., Santana, A. & Pedraza-Alva, G. CD43, a molecule with multiple functions. Immunol. Res.20, 89–99 (1999). ArticleCASPubMedGoogle Scholar
- Sunderkotter, C. et al. Subpopulations of mouse blood monocytes differ in maturation stage and inflammatory response. J. Immunol.172, 4410–4417 (2004). ArticlePubMedGoogle Scholar
- Yrlid, U., Jenkins, C. D. & MacPherson, G. G. Relationships between distinct blood monocyte subsets and migrating intestinal lymph dendritic cells in vivo under steady-state conditions. J. Immunol.176, 4155–4162 (2006). ArticleCASPubMedGoogle Scholar
- Briseno, C. G. et al. Distinct transcriptional programs control cross-priming in classical and monocyte-derived dendritic cells. Cell Rep.15, 2462–2474 (2016). ArticleCASPubMedPubMed CentralGoogle Scholar
- Anderson, K. G. et al. Intravascular staining for discrimination of vascular and tissue leukocytes. Nat. Protoc.9, 209–222 (2014). ArticleCASPubMedPubMed CentralGoogle Scholar
- Hildner, K. et al. Batf3 deficiency reveals a critical role for CD8α + dendritic cells in cytotoxic T cell immunity. Science322, 1097–1100 (2008). ArticleCASPubMedPubMed CentralGoogle Scholar
- Murphy, K. M. Transcriptional control of dendritic cell development. Adv. Immunol.120, 239–267 (2013). ArticleCASPubMedGoogle Scholar
- Jakubzick, C. & Randolph, G. J. Methods to study pulmonary dendritic cell migration. Methods Mol. Biol.595, 371–382 (2010). ArticlePubMedGoogle Scholar
- de Heer, H. J. et al. Essential role of lung plasmacytoid dendritic cells in preventing asthmatic reactions to harmless inhaled antigen. J. Exp. Med.200, 89–98 (2004). ArticleCASPubMedPubMed CentralGoogle Scholar
- Mitchell, L. A., Hansen, R. J., Beaupre, A. J., Gustafson, D. L. & Dow, S. W. Optimized dosing of a CCR2 antagonist for amplification of vaccine immunity. Int. Immunopharmacol.15, 357–363 (2013). ArticleCASPubMedGoogle Scholar
Acknowledgements
The authors acknowledge support from the following National Institutes of Health grants: R01HL115334 (to C.V.J.), R37AI049653 (to G.J.R.) and R01HL114381 (to P.M.H.). They also thank T. D. Wager for carefully reading the manuscript.